- Basic Concepts of Electrostatics
- How does electricity work?
- Faraday’s Laws of Electromagnetic Induction
- Ideal & Practical Voltage Sources
- Internal Resistance of Electric Source
- Ohm’s Law and Concept of AC & DC
- What is Peak, R.M.S. & Average Value of ac voltage and current
- Kirchhoff’s Current Law (KCL)
- Kirchhoff’s Voltage Law (KVL)
- Concept of Electric Power
- Concept of AC voltage – Learn in simple language
- What are the types of electric currents? Explain with diagram
Key Contributors
It is very interesting to know first about the key contributors to the concept of electric current.
William Gilbert (1544–1603)
Often considered the “father of electricity,” he studied magnetic and electric phenomena and coined the term “electricus” to describe the force of attraction observed in rubbed materials like amber.
Benjamin Franklin (1706–1790)
Conducted experiments with electricity and introduced the concept of positive and negative charges. He described electric current as the flow of a “fluid,” though his understanding was limited to static electricity.
Alessandro Volta (1745–1827)
Invented the Voltaic Pile, the first chemical battery, in 1800. This provided a steady flow of electric current for the first time and paved the way for studying current electricity.
André-Marie Ampère (1775–1836)
Known as the “father of electrodynamics,” he formulated the mathematical theory of electric current and described the relationship between current and magnetic fields. The unit of electric current, the ampere, is named after him.
Georg Simon Ohm (1789–1854)
Discovered the relationship between voltage, current, and resistance, now known as Ohm’s Law.
Michael Faraday (1791–1867)
Demonstrated the connection between electricity and magnetism through electromagnetic induction, which showed how electric currents could be generated.
The concept of electric current as a flow of charged particles (electrons) was fully understood later, particularly with the development of atomic theory in the 19th and early 20th centuries. The discovery of the electron by J.J. Thomson in 1897 clarified the microscopic nature of electric current.
Types of Electric Current
There are 3 types of electric currents –
- Steady Current
- Varying Current
- Alternating Current
Now let us discuss each of them in simple language.
Steady Current
A steady current maintains a constant magnitude over time, making it predictable and consistent. In other words, its value does not fluctuate as time progresses. This type of current is represented graphically in following figure, where the current remains unchanged despite the passage of time. A common example of steady current, also known as direct current (DC), is the current supplied by a battery.
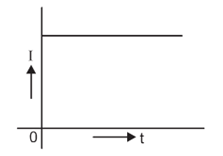
Varying or Changing Current
Unlike steady current, the magnitude of a varying current changes with time. This type of current does not maintain a consistent value and instead fluctuates. The following figure illustrates a graph of varying current, showing how the current value rises and falls over time. Such currents are often observed in dynamic systems where the load or supply conditions keep changing.
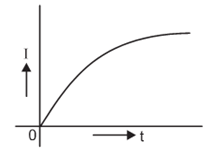
Alternating Current
Alternating current (AC) is unique in that its magnitude continuously varies with time and its direction reverses periodically. This periodic change gives AC its characteristic waveforms, typically sine or cosine waves, as shown in following figure. For one half of the cycle (0 to T/2 seconds, where T is the time period), the current flows in one direction, and during the other half (T/2 to T seconds), it flows in the opposite direction. Alternating current is widely used for its technical and economic advantages and is commonly generated by AC generators.
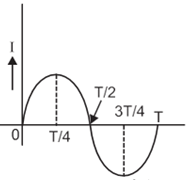
- Steady Current: Consistent and unchanging, like the current from a battery.
- Varying Current: Dynamic and fluctuating in magnitude.
- Alternating Current: Cycles back and forth, with a sine wave pattern, used in most power systems.
This classification helps us understand how electric currents behave under different conditions and their practical applications in everyday devices.
Motion of Electrons in Metal
Now let us see in details about the motion of electrons in metals.
Free Electrons
Every metal is filled with a large number of free electrons, which move randomly within the conductor much like gas molecules. At room temperature, these electrons have an average speed of approximately 10⁵ m/s. However, this seemingly chaotic motion doesn’t result in a net flow of charge because the electrons collide frequently with the metal’s positively charged ions. Each collision alters their direction of motion, and the overall effect of these random movements averages to zero. As a result, there is no net current in the conductor under normal conditions.
Application of Electric Potential
When a potential difference is applied across the ends of a metallic conductor, an electric field is created inside it. This field exerts a force on the free electrons, causing them to accelerate towards the positive terminal (opposite to the direction of the electric field).
As these electrons move, they continue to collide with the positive ions of the metal. Each collision reduces the additional velocity gained due to the electric field. This interplay between acceleration and collisions leads to a net, slow movement of electrons in the direction of the positive terminal. This slow, average motion of electrons is called the ‘drift velocity’.
Drift Velocity and Current Flow
Although the individual speed of free electrons is incredibly high, their drift velocity under the influence of an electric field is remarkably small—of the order of 10⁻⁵ m/s. Despite its small value, this drift velocity is what makes the flow of electric current possible in a conductor.
To visualize this, imagine turning on a light. The light seems to illuminate instantly, even though the electrons themselves drift very slowly. This is because the electric field propagates through the conductor at nearly the speed of light, setting the electrons in motion almost simultaneously along the wire.
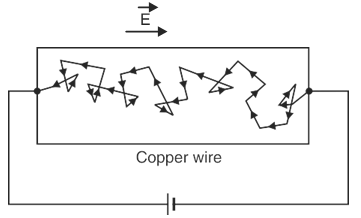
The Role of Collisions
During their journey through the conductor, free electrons continuously collide with the positive ions of the metal. These collisions prevent the electrons from gaining excessive velocities and ensure that their motion remains steady under the applied electric field. The time between two successive collisions, known as ‘relaxation time,’ is of the order of 10⁻¹⁴ seconds.
These frequent collisions, combined with the applied electric field, give rise to the steady flow of current in the conductor. This balance of forces creates a uniform drift velocity, making the conduction process efficient and predictable.